Our Research Based on:
Making the World Smarter and Better
1 hr
220 US dollars1 hr
150 US dollars1 hr
170 US dollars
1. Multi‐Functional Biomaterials for Drug/Gene Delivery
Enzymes are nature approved the most efficient biocatalysts that could enable faster reaction with more energy efficiency. Unlike another conventional chemical catalyst, the biocatalyst is biodegradable and function at mild conditions in water with higher selectivity. The enzyme has been widely used to convert chemical to useful fuels and drugs, kill disease, detoxify nerve agent and treat environmental contamination. However, enzymes cannot always compete economically with traditional chemistry and inorganic catalysts. The major challenges preventing widespread use of enzymatic biocatalysis are the cost of production, low loading, limited stability, and separation difficulties. For commercial‐scale processes, enzymes are often immobilized on solid supports so that they can be reused. If done effectively, though, immobilization can be advantageous. For example, they can tolerate organic solvents and higher temperatures and can be used and reused efficiently over multiple synthesis cycles.
We aim to develop new assembly strategies with molecular‐level control of the soft interface between polymer and enzyme, thereby enabling the design of truly integrated, multifunctional bio‐hybrid hierarchical enzyme systems with remained activity, enhanced stability, better reusability (Figure 1). We plan to master these hierarchical structures by fine‐tuning the interactions between polymer and enzyme chemically and structurally. A fundamental understanding of the molecular‐level interaction during the formation of these hierarchical structures from in situ X‐ray scattering at Advanced Photon Source (APS) and in situ transmission electron microscopy (TEM) at the center of nanoscale materials (CNM) of Argonne National Laboratory (ANL) and structural insights of the enzymes can provide basic science guidance to develop a transformative synthesis for these hybrid polymer–enzyme nanoparticles (NPs).
2. High‐Performance Nano‐Catalysts for Energy Conversion
Coking and sintering are two main methods of deactivating the catalysts consisting of metal nanoparticles (NPs) on the supports. Coking is caused by the accumulation of carbon on the metal surface that can block the metal catalysts while sintering is from the formation of larger metal particles that can reduce the overall surface area and reactivity. This deactivation is costly and can limit the development of novel catalysts. Many efforts have been made to address these two problems, but most approaches still focus on one issue individually. several methods have been achieved while simultaneously inhibiting the coking and sintering of supported metal catalysts, also maintaining high catalytic activity for high-temperature applications.
Recently, coking and sintering can be effectively suppressed by “overcoating” the noble metal NPs with metal oxide layers using atomic layer deposition (ALD). The overcoat initially fully encapsulates the nanoparticle, but after being calcined at high temperatures, porosity forms in the ALD overcoat, re‐exposing the underlying metal. The dimensions of these nanopores can be tuned to impart shape selectivity: only reactants or products with a specific size or shape can fit inside the pore. However, the details of this pore formation process are unknown. Understanding the mechanisms of the pore formation is desirable because it may provide the necessary insights that can guide the generation of highly controllable ALD overcoated catalysts. One reason why pore formation has not yet been conclusively understood is due to the limitations of traditional methods such as Brunauer–Emmett–Teller (BET) and Barret–Joyner–Halenda (BJH) for in situ characterization of porous materials. In addition, transmission electron microscopy (TEM) provides limited information due to the low contrast between NPs and pores, and it is difficult to perform in situ TEM measurement of the catalytic systems. Therefore, the application of time‐resolved techniques capable of characterizing and in situ probing the evolution of pore formation is of great importance. Using time‐resolved synchrotron X‐ray techniques are promising X‐ray candidates for real‐time monitoring of pore formation and evolution. Among them, simultaneous small‐ and wide-angle X‐ray scattering (SAXS/WAXS) has been demonstrated to be a very powerful technique for studying the growth of nanoparticle and a variety of nanostructures such as colloid, porous materials, biomolecules, and proteins, ranging in size from 1 to 200 nm. Such work is supported by NSF and DOE.
3.Investigating the Solvation Behavior of Electrolytes for Battery Materials
The solubility of electrolyte components plays a critical role in energy storage and hence the ability to predict, regulate and engineer solubility is imperative. For example, the solubility of electrochemically active species sets the limit of the redox-flow energy density, the conductivity and transference number of multivalent electrolytes, and the reactivity of Li-S systems. Moreover, recent discoveries show that in many solution systems, nano-sized structural units are present below the solubility limit. However, little is known about the structure and role of these clusters in solution and no predictive framework is available. Similarly, electrolyte transport properties, such as ionic conductivity and transference number, are critical to the performance of energy storage systems. Recent investigations conducted outside the regimes of dilute behavior show that carefully designed solvation structures, even at very high concentrations, can enable unique electrolyte behavior along with acceptable ionic conductivities. Organizational structures of the active species, such as spatially extended percolating networks, can provide a path that assists ion hopping over distance through the network. Detailed understanding is needed to harness such unique solvation structures across monovalent to multivalent electrolytes and to elucidate their longer-range impact on ion transport. We propose to systematically study the effect of ionic size, charge, and composition on how solvation phenomena and the resulting clustering behavior regulate solubility limits across a range of electrolyte classes, including those containing divalent cations and redoxmer species. In addition to characterizing solvation with respect to first/second shell interactions, we will utilize X-ray and neutron scattering techniques to describe longer-range solution organization. Microscopic ion transport mechanisms will be probed over a range of length scales from several to tens of nanometers with X-ray Photon Correlation Spectroscopy utilizing APS facilities, which measures the correlated or collective motion of conducting ions and the surrounding molecules through time-dependent speckle-patterns in the diffused and small-angle scattering regime.
4. Advanced Characterization Tools for In Situ and Operando Characterization of Energy Materials
Understanding solutions and materials chemistry requires knowledge of structure at the atomic level. Most of the standard structural characterization tools provide high‐quality information on bulk, periodic, or crystalline materials. Equally, high‐quality characterization is needed for amorphous materials, solutions, and complex nanostructures. Synchrotron‐based techniques can now be used to characterize samples irrespective of their state of crystallinity via different scattering and spectroscopic methods. X‐rays are providing structural details at length scales that encompass local structure about an atom to the longer-range ordering up to about 10 nm and have found applications in liquids, poor‐quality crystals, multiphase powders, assemblies of micro‐crystals, adsorbed surface layers, etc. Moreover, the evolution of structure under reaction conditions is also an important feature of a catalytic system thus each method needs to provide this information under a wide ran of conditions, e.g., gas, liquid, solid including high pressure and temperature. We have effectively utilized in situ and operando X‐ray absorption spectroscopy (EXAFS and XANES), small-angle scattering (SAXS), RAMAN, and electron microscopies to solve structural details relevant to catalysis and battery.
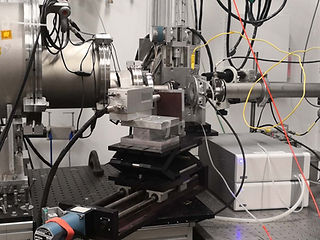


